Written by Marine Daïeff
Published April 8th 2020
Contact: partnership@elvesys.com, Elvesys SAS, 172 Rue de Charonne 75011 Paris
Acoustic techniques and their use in microfluidic devices (acoustofluidics) are presented in this review as a way to separate and sort particles in microfluidic systems. For further information we recommend referring to the interesting reviews from Lenshof et al, 2012, Sajeesh et al, 2013, and Xi et al, 2017.
For label-free passive particle sorting, we assembled a particle size sorting beta pack.
1. Introduction to acoustic techniques
Thanks to the development of microfabrication and microfluidic devices, standard techniques to separate and sort particles depending on their properties such as shape, flexibility and activity have been developed during the past decades. The scale of microfluidic systems is of great interest in medical, biological and food sciences with applications such as diagnostics, chemical and biological analysis. Microfluidic systems can integrate various sorting methods in regards to the physical properties of the particles but can also be used with external fields such as acoustic, optical and magnetic. The use of acoustic waves to sort particles from all sizes is particularly efficient, versatile and biocompatible.
Figure 1: Acoustic waves enable the separation of particles with different sizes (Lenshof et al, 2012).
2. Different waves used for acoustofluidics in microfluidic devices
Two different wave modes are often used in order to sort particles: Surface Acoustic Wave (SAW) and Bulk Acoustic Wave (BAW). When the waves interact with the medium, two acoustic phenomena appear: acoustic streaming and acoustic radiation force. They act on the particles inside the microfluidic channels and it is either one of these phenomena or a combination of them which is used to sort particles. The waves are generally created from interdigitated transducers (IDT) comprising electrodes placed on the sides of the channel. The SAW is unique and travels on the surface of a substrate. Most of the time, half-wavelength resonators are used. In this case, the pressure node corresponding to a minimum pressure amplitude is located at the center of the microfluidic channel while the antinode corresponding to a maximum pressure amplitude is located on the channel wall itself. Two different SAWs, respectively a Traveling Surface Acoustic Wave (TSAW) and a Standing Surface Acoustic Wave (SSAW) are reported depending on the way they are generated, using either a single set of IDTs or two different sources.
The BAW is a wave propagating in the medium generally generated by bulk piezoelectric transducers instead of IDTs. The transducer is typically placed underneath the microfluidic device. In order to optimize the global performance of the methods, a close study needs to be carried out to choose the best range of frequencies and the most accurate geometry for the microfluidic devices. In fact, the efficiency of the acoustofluidic method strongly depends on the size of the particles studied and the physical properties of the fluid used. It is also important to clarify that acoustic techniques are best suited for a size range of particles from a micron to 10-20 microns. More detail on the acoustic waves can be found in Xi et al, 2017. Most of the experiments presented in the following review have been performed using SAWs.
Figure 2: Schematic summarizing the different acoustic waves used to sort particles. Orange stands for oil flow and green stands for aqueous flow (Xi et al, 2017).
3. Sorting particles in microfluidic devices depending on their size: free-flow acoustophoresis
In free-flow acoustophoresis, particles are basically driven towards a pressure node by acoustic radiation force while transported with the flow. It can be shown that this acoustic radiation force is proportional to the third power of the radius of the particle (Lenshof et al, 2012). Therefore, the bigger the particle, the stronger the force acting on the particle and then the faster it is moving to the pressure node (Figure 3a).
Free-flow acoustophoresis is therefore a good method for multiple fractionation (Figure 3b). A solution of mixed particles beforehand focused is exposed to the acoustic force. The bigger particles move faster to the pressure nodes compared to smaller ones. As a result, the different-sized particles are on different positions in the microfluidic channel. With as many outlets as size of particles, it is then possible to sort the particles in an easy way. In order to design efficient microfluidic devices, two parameters need to be taken care of: the width of the particle stream when it enters the acoustic zone compared to the channel width and the distance travelled by the particles in the acoustic zone. Free-flow acoustophoresis is popular in medical applications. For example, it has been used to separate leukocytes, red cells and platelets (Petersson et al, 2007). For highly concentrated solutions, the common configuration of a half wavelength acoustic resonator followed by a splitter is not efficient enough. Some specific designs have been reported, where particles are sequentially removed from the main flow in the center of the channel. This technique enables the development of plasmapheresis and important improvements have been made to remove blood cells in order to obtain clarified blood plasma (Lenshof et al, 2009).
Figure 3: Sorting particles in microfluidic devices using free-flow acoustophoresis: (a) separation based on size (reported by Johnson and Feke, 1995), (b) multiplexed separation of mixed suspension through a two steps process (Guldiken et al, 2012).
[starter_pack_rebound]
4. Sorting particles in microfluidic devices depending on their acoustic contrast factors: binary acoustophoresis
Besides size, differences in density and compressibility can be utilized to separate and sort particles. These two parameters are indeed involved in the calculation of the acoustic contrast factor of the particles (Lenshof et al, 2012). The separation process called binary acoustophoresis is based on the idea that two particles may have different acoustic contrast factors. Particles with positive acoustic factors are driven to the pressure nodes by the primary acoustic force. On the contrary, for particles with negative acoustic factors, the force acts in the opposite direction and particles move to antinodes. For half-wavelength resonators, particles are separated between the center outlet and side outlets.
It is then possible to separate and sort particles (cells encapsulated) with the same size but different densities (Figure 4a). The number and density of cells encapsulated modify the density of the beads. After focusing the beads in the center of the channel by the application of a sheath flow, the beads drift laterally when the acoustic wave is applied depending on their density. The deflection is maximal for the beads of large density; no deflection is observed for the beads with no cell. Particles of polymer with comparable size and density can be sorted by binary acoustophoresis because of different compressibility (Figure 4b). Binary separation is of great interest for applications in the medical field such as removing lipid emboli from shed blood during cardiac surgery (Petersson et al, 2004) or food field (Figure 4c). In some situations, particles studied have comparable acoustic contrast factors. However, a solution is to change the buffer in order to get particles with opposing polarities (Gupta et al, 1995). A binary separation can then be performed by adjusting the density of the medium adding cesium chloride for example (Petersson et al, 2007). In order to find such solutions, it is required to know the acoustophysical properties of the species studied. Developments have recently been made to measure compressibility and density of particles (Augustsson et al, 2011).
Figure 4: Sorting particles in microfluidic devices using binary acoustophoresis: (a) separation of beads based on density (Nam et al, 2012), (b) separation of polymer particles based on compressibility (Gupta et al, 1995), (c) separation of lipid particles (white) in milk driven by different acoustic contrast factors (Grenvall et al, 2009).
5. Sorting particles in microfluidic devices by manipulating frequencies of acoustic waves
By switching frequencies of acoustic waves and as a result, by tuning the magnitude of the primary acoustic radiation force, it is possible to obtain an alignment of two different particles into different pressure nodes (Figure 5a). An excellent control over the frequencies and the intervals of time is required in order to get the best results, as well as a good control over the temperature. Switching frequencies is also a good technique to sort similar particles to multiple outlets. Particles are driven to different pressure nodes according to the frequency applied. The channel design and the choice of frequencies need to be carefully chosen in order to optimize the set up (Figure 5b). In order to sort a population of a narrow size distribution, it is possible to connect several resonators to obtain a “band-pass” particle size filtering (Figure 5c). The frequencies of the two acoustophoretic zones can be tuned separately and independently, allowing a better control over the separation.
Figure 5: Sorting particles in microfluidic devices by manipulating frequencies of acoustic waves: (a) particles of different sizes are separated by modifying the position of acoustic nodes in the microfluidic channel (Liu and Lim, 2012), (b) identical water-in-oil droplets are sorted to different outlets by tuning the frequency of the acoustic wave (Li et al, 2013), (c) acoustic tunable device called acoustic “band-pass” particle sorter filtering particles by size (Adams and Soh, 2010).
[seqinject_pack_rebound]
6. Medium/buffer switching in microfluidic devices using acoustic waves
Finally, using acoustic waves is also a good way to change particles from a solution to another. The flows in microfluidic channels are laminar. It is then possible to have two laminar streams flowing parallel to each other in a microfluidic channel. By applying an acoustic wave, it is possible to move particles from one stream to the other. The medium/buffer switch is equivalent to a centrifugal wash. Different designs to complete the medium/buffer switch have been reported: a layered resonator (Figure 6a), a microfluidic channel with two inlets and two outlets (Figure 6b), a transversal resonator (Figure 6c) and a device with several inlets and outlets located on the side of the channel (Figure 6d).
The use of acoustophoresis reduces the analysis time and the risk of errors as several centrifugation steps are not needed any more. This technique is also of great interest in order to pull out rare species present in complex solutions. It is possible to use beads with particular affinity with the particles selected, and after binding them it is possible to sort out the selected species (Persson et al, 2008).
Figure 6: Different principles of medium switching in microfluidic devices using acoustic waves: (a) a layered resonator where the aim is to elevate the particles in the upper channel (Hawkes et al, 2004), (b) a separation process of two species using a microchannel with two inlets and two outlets (Liu et al, 2012), (c) a transversal resonator where particles are focused in the center of the channel in the clean buffer (Petersson et al, 2005), (d) an acoustic washing device with several inlets and outlets located on the side of the channel (Augustsson et al, 2009).
SUMMARY
Cells and other bioparticles can be isolated with high yield, purity, and biocompatibility using acoustofluidic separation – a powerful tool due to the label-free, biocompatible, and contact-free nature of the technology. The development of automated, point-of-care devices for isolating sub-micron bioparticles has been made possible by carefully designing and tuning the applied acoustic field. Thus, many of the limitations of conventional separation tools can be overcome. In addition, advances in the research lab can quickly be adopted to solve clinical problems (Wu et al., 2019).
References
- Lenshof A., Magnusson C., Laurell T., Acoustofluidics 8: Applications of acoustophoresis in continuous flow microsystems, Lab Chip, 2012, 12, 1210, DOI: 10.1039/c2Ic21256k
- Sajeesh P., Sen A.K., Particle separation and sorting in microfluidic devices: a review, Microfluidics and Nanofluidics, 2013, 17(1), 1-52, DOI: 1007/s10404-013-1291-9
- Xi H.-D., Zheng H., Guo W., Ganan-Calvo A. M., Ai Y., Tsao C.-W., Zhou J., Li W., Huang Y., Nguyen N.-T., Tan S. H., Active droplet sorting in microfluidics: a review, Lab Chip, 2017, 17, 751, DOI: 10.1039/c6lc01435f
- Petersson F., Aberg L., Sward-Nilsson A.-M., Laurell T., Free Flow Acoustophoresis: Microfluidic-Based Mode of Particle and Cell Separation, Chem., 2007, 79(14), 5117-5123, DOI: 10.1021/ac070444e
- Lenshof A., Ahmad-Tajudin A., Järas K., Swärd-Nilsson A.-M., Aberg L., Marko-Varga G., Malm J., Lilja H., Laurell T., Acoustic Whole Blood Plasmapheresis Chip for Prostate Specific Antigen Microarray Diagnostics, Chem., 2009, 81(15), 6030-6037, DOI: 10.1021/ac9013572
- Johnson D. A., Feke D. L., Methodology for fractionating suspended particles using ultrasonic standing wave and divided flow fields, Separations Technology, 1995, 5(4), 251-258, DOI: 10.1016/0956-9618(95)00130-1
- Guldiken R., Jo M.C., Gallant N.D., Demirci U., Zhe J., Sheathless Size-Based Acoustic Particle Separation, Sensors, 2012, 12(1), 905-922, DOI: 10.3390/s120100905
- Petersson F., Nilsson A., Holm C., Jonsson H., Laurell T., Separation of lipids from blood utilizing ultrasonic standing waves in microfluidic channels, Analyst, 2004, 129(10):938-943, DOI: 10.1039/b409139f
- Gupta S., Feke D. L., Manas-Zloczower I., Fractionation of mixed particulate solids according to compressibility using ultrasonic standing wave fields, Eng. Sci., 1995, 50(20), 3275-3284, DOI: 10.1016/0009-2509(95)00154-w
- Augustsson P., Barnkob R., Wereley S. T., Bruus H., Laurell T., Automated and temperature-controlled micro-PIV measurements enabling long-term-stable microchannel acoustophoresis characterization, Lab Chip, 2011, 11(24), 4152-4164, DOI: 10.1039/C1LC20637K
- Nam J., Lim H., Kim C., Kang J.Y., Shin S., Density-dependent separation of encapsulated cells in a microfluidic channel by using a standing surface acoustic wave, Biomicrofluidics, 2012, 6(2):024120, DOI: 10.1063/1.4718719
- Grenvall C., Augustsson P., Folkenberg J. R., Laurell T., Harmonic Microchip Acoustophoresis: A Route to Online Raw Milk Sample Precondition in Protein and Lipid Content Quality Control, Chem., 2009, 81, 15, 6195-6200, DOI: 10.1021/ac900723q
- Liu Y., Lim K.-M., Particle separation in microfluidics using a switching ultrasonic field, Lab Chip, 2011, 11, 3167-3173, DOI: 10.1039/C1LC20481E
- Li S., Ding X., Guo F., Chen Y., Lapsley M. I., Lin S.-C. S., Wang L., McCoy J. P., Cameron C. E., Huang T. J., An On-Chip, Multichannel Droplet Sorter Using Standing Surface Acoustic Waves, Chem., 2013, 85, 11, 5468-5474, DOI: 10.1021/ac400548d
- Adams J.D., Soh H.T., Tunable acoustophoretic band-pass particle sorter, Phys. Lett., 2010, 97, 064103, DOI: 10.1063/1.3467259
- Persson J., Augustsson P., Laurell T., Ohlin M., Acoustic microfluidic chip technology to facilitate automation of phage display selection, FEBS, 2008, 275, 5657-5666, DOI: 10.1111/j.1742-4658.2008.06691.x
- Hawkes J. J., Barber R. W., Emerson D. R., Coakley W. T., Continuous cell washing and mixing driven by an ultrasound standing wave within a microfluidic channel, Lab Chip, 2004, 4, 446-452, DOI: 10.1039/B408045A
- Liu Y., Hartono D., Lim K.-M., Cell separation and transportation between two miscible fluid streams using ultrasound, Biomicrofluidics, 2012, 6: 012802, DOI: 10.1063/1.3671062
- Petersson F., Nilsson A., Jonsson H., Laurell T., Carrier Medium Exchange through Ultrasonic Particle Switching in Microfluidic Channels, Chem., 2005, 77, 5, 1216-1221, DOI: 10.1021/ac048394q
- Augustsson P., Aberg L. B., Sward-Nilsson A.-M. K., Laurell T., Buffer medium exchange in continuous cell and particle streams using ultrasonic standing wave focusing, Microchim Acta, 2009, 164, 269-277, DOI: 10.1007/s00604-008-0084-4
- Wu, M., Ozcelik, A., Rufo, J. et al., Acoustofluidic separation of cells and particles, Microsystems & Nanoengineering, 2019, 5, 32, https://www.nature.com/articles/s41378-019-0064-3
This review was written by Marine Daïeff – Research & Innovation Engineer in Microfluidics, Elvesys – Microfluidics innovation center.
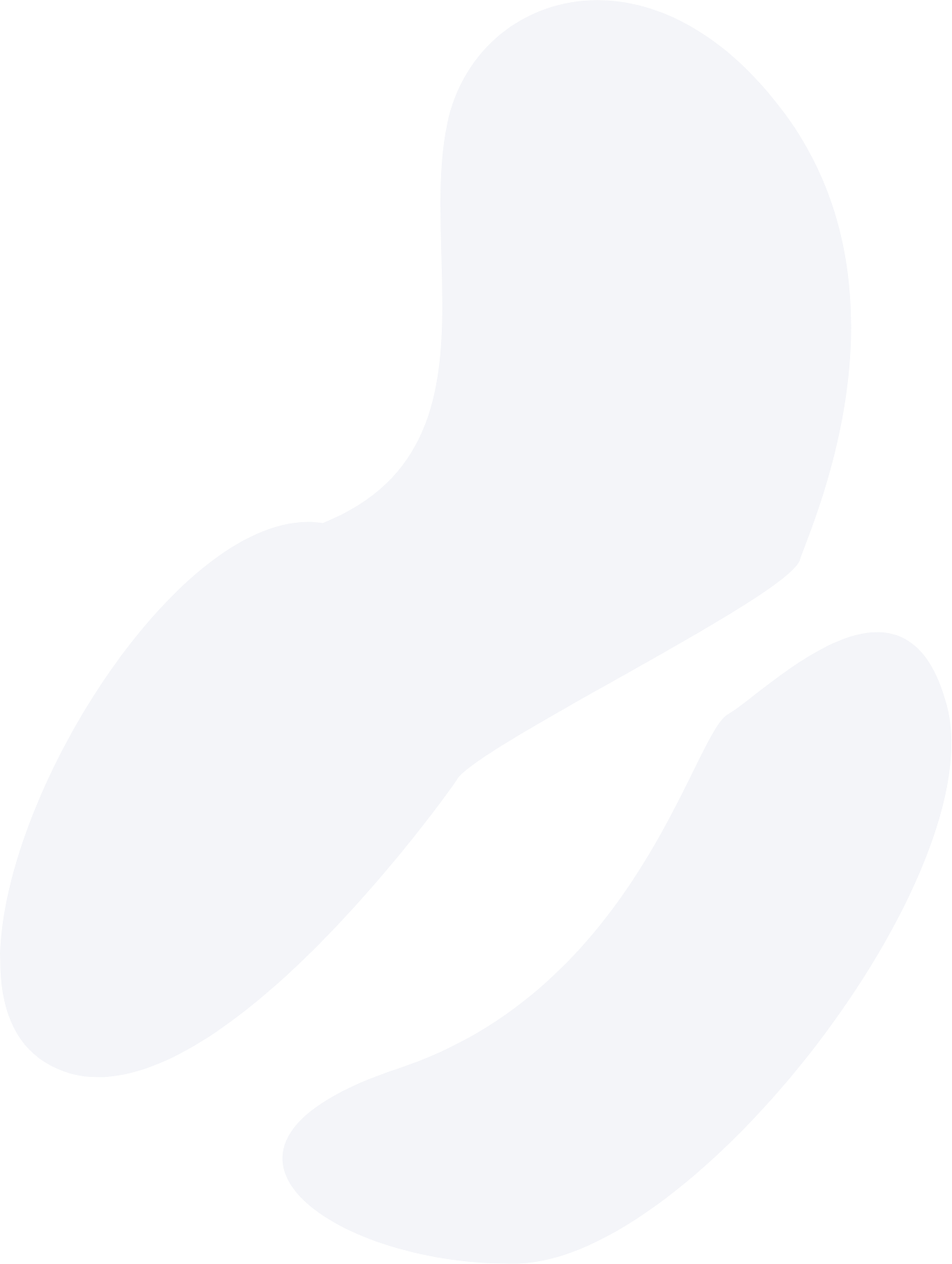
Microfluidics knowledge
Do you want tips on how to best set up your microfluidic experiment? Do you need inspiration or a different angle to take on your specific problem? Well, we probably have an application note just for you, feel free to check them out!