Written by Audrey Nsamela
Published April 22th 2021
Contact: partnership@elvesys.com, Elvesys SAS, 172 Rue de Charonne 75011 Paris
Introduction to Microfluidics for sperm sorting
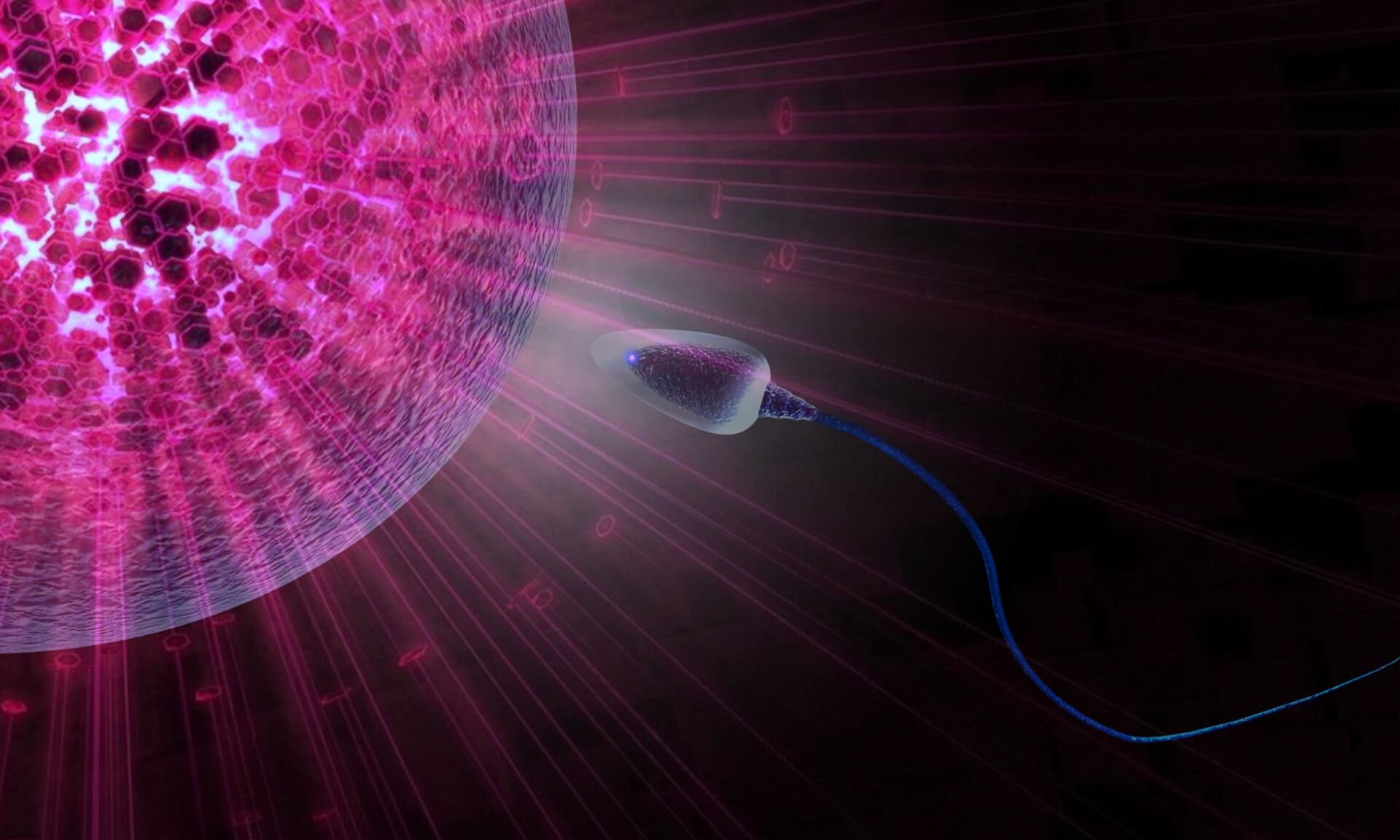
Assisted reproductive technologies (ARTs) are very important tools to deal with infertility problems which affect almost 50 million couples around the world. It is estimated that about half of couples’ infertility cases are attributed to male infertility, hence poor sperm quality or insufficient concentration of spermatozoa. This is why sperm selection is crucial for its use in ARTs such as in vitro fertilization (IVF), intra-cytoplasmic sperm injection (ICSI) and intrauterine insemination (IUI).
Sperm sorting can be made based on different criteria such as motility, surface chemistry, morphology or sex. As it has been merely twenty years since the potential of microfluidics has been recognized for ARTs, findings in this emergent field have been more abundant in the motility based selection method [2]. However, human infertility is not the only area where sperm sorting is applicable, it is also useful in the dairy industry with gender-based sperm sorting (also called sexing). Indeed, by inducing a gender bias towards female calves before their birth we can prevent unethical disposal of “unwanted” male calves. Recently, the benefits of sperm sexing have also been applied for the conservation of endangered species [3]. Sexing solutions are commercially available and are based on different approaches: flow cytometry, electrophoresis and photonic-based techniques. This review does not intend to be comprehensive on that matter and will focus more on size and motility-based selection.
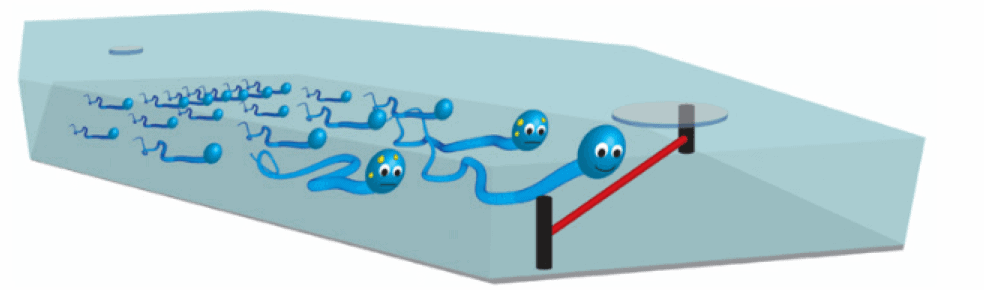
Figure 1 – Illustration of sperm selection in microfluidics [1].
During natural fertilization, spermatozoids are ejaculated in seminal plasma into the vagina, close to the entrance of the cervix and have to travel through highly viscous fluids and narrow passages all the way to the egg. Conventional techniques to select the best fitted sperms are usually centrifugation-based and are not inspired by the natural selection process. The most famous ones are the swim up, density gradient centrifugation and migration-sedimentation techniques [4]. These techniques, although widely used in clinics and accepted by the community, were proved to be harmful to the sperm cells as they induce the production of reactive oxygen species (ROS) which leads to a loss of DNA integrity in the sample [2], [4]. The centrifugation steps exert large forces on the spermatozoids and the time required for the sorting is usually quite long. These limitations have driven the search for new technologies for sperm selection. Microfluidics provides the appropriate tools to solve issues with current ARTs, from sperm selection to artificial fertilization, in a more democratic and less invasive way. Microfluidic systems are also able to mimic closely in vivo conditions of sperm selection [5].
Microfluidics for sperm sorting: passive selection
Passive selection does not refer to “passively driven” microfluidics but to separation methods based on parameters others than the motility of the cells, e.g., their size and density. In the case of sperm selection, passive selection based on size is highly relevant when processing raw semen. Indeed, sperm cells are usually isolated from the seminal plasma and other types of cells (e.g., red blood cells and macrophages) by centrifugation and washing steps. Although the presence of these cells is not proven to be damaging to the sperm quality or the outcome of IVF or ICSI, in some cases the concentration of these cells is too high compared to the concentration of motile sperm cells. Hence, researchers developed a method to efficiently separate motile sperm cells from red and white blood cells in raw semen samples with a microfluidic device,allowing the purification of semen samples while retaining a maximum number of motile sperm cells and preserving the original fertilization capacity [6]. This cell sorting technique is based on the fact that cells with different sizes and density will experience inertial forces with different intensity when flowing in a spiral microchannel: bigger cells tend to migrate to the outer rim of the channel while smaller cells will remain closer to the center of the channel, depicted in figure 2. This sorting device is not cell specific and can also be used to sort microparticles. For more information see this cell separation and sorting review. Due to its versatility, the spiral microchannel chip is available commercially and Microfluidic chip shop Gmbh is fabricating them in various materials using injection molding technology. See this review for additional information on the different materials suitable for the fabrication of microfluidic chips.
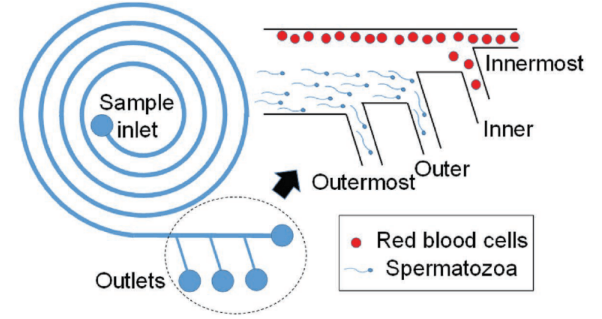
Figure 2 – Schematic illustrating the principle of a microfluidic spiral sorter [5].
Microfluidics for sperm sorting: active selection
Active selection means a set of strategies using the active swimming of the sperm cells. These techniques take their inspiration from the natural selection that occurs in the female reproductive tract based on mainly 3 phenomena: chemotaxis, thermotaxis and rheotaxis. The term taxis refer to an active migration of cells in response to a stimulus, the nature of this stimulus will then determine the prefix of the word as defined hereafter.
Chemotaxis
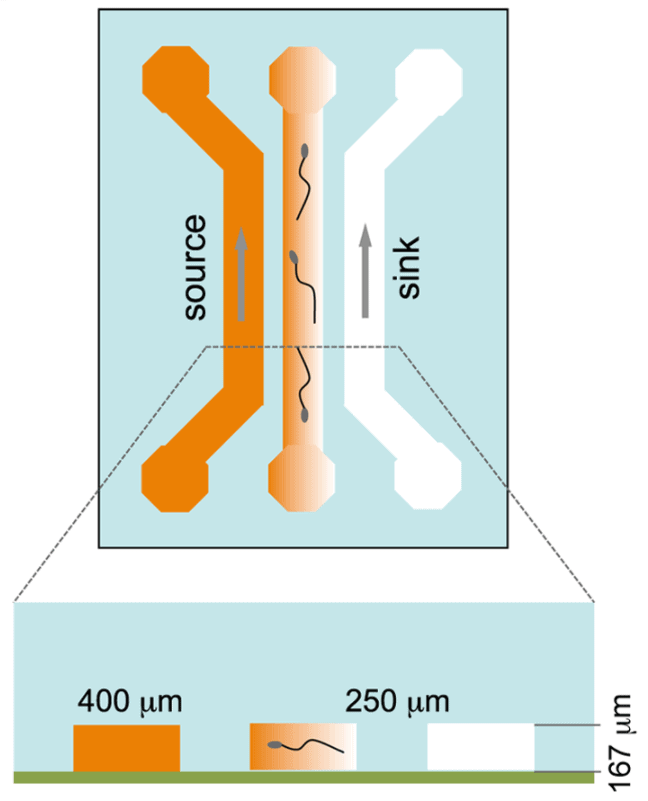
Figure 3 – Microfluidic device for gradient generation to study chemotaxis in mouse sperm [20].
Chemotaxis is defined as the movement of a microorganism, or an artificial swimmer, towards or away from a chemical called a “chemoattractant”. Many cells, whether they are motile or not, are sensitive to chemical gradients and so are sperm cells. Chemotaxis is one of the mechanisms that is believed to have a very important role in the spermatozoa’s guidance towards the egg. Indeed, when placed in a concentration gradient of certain molecules, sperm cells naturally swim towards the most concentrated areas. In humans, cumulus cells present around the egg secrete a well-known hormone: progesterone. This hormone is commonly used as a chemoattractant for chemotaxis assays of human sperm cells. Microfluidics allows for controlled establishment of chemical gradients and a wide variety of designs are available for these so-called gradient generators. One design in particular is famous for its ability to generate a transverse gradient in a straight flow-free microchannel and was used to perform a chemotaxis assay of mouse sperm cells (see figure 3). The central microchannel is surrounded by two parallel channels called the “source” and the “sink”, each separated with a semi-permeable hydrogel.
The chemoattractant is then injected in the source microchannel and diffuses through the hydrogel to the central microchannel containing the spermatozoa. Although chemotaxis is systematically mentioned as a natural means of sperm selection, only a few studies using this method to successfully sort sperm cells in microfluidics are available [7], [8].
Thermotaxis
The definition of thermotaxis is very similar to the one of chemotaxis except that in this case, as the name suggests, the driving force is a thermal gradient rather than a chemical gradient. Contrarily to chemotaxis, thermotaxis is a relatively long range mechanism, as depicted in figure 4, and sperm cells are among the most sensitive motile cells to thermal gradients [9]. Human sperm cells have been found to become hyperactivated and swim up thermal gradients characterized by a temperature difference of only a few degrees Celsius [10]. Proof-of-concept microfluidic platforms for sperm selection have been successfully realized with mice and human sperm [8], [11]. In a particular study performed by Pérez-Cerezales et al., thermotaxis on chip sperm selection significantly improved the outcome of ICSI in mice, more than doubling the number of pregnancies as compared to sperm cells selected by the conventional swim up method [11].
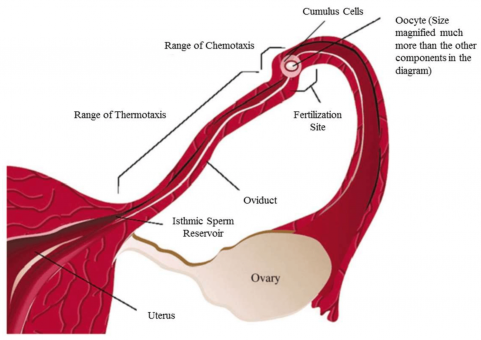
Figure 4 – Illustration of the fertilization site in the female reproductive tract and the range of thermotaxis and chemotaxis [9].
[ob1f_rebound]
Flow and rheotaxis
Flow and rheotaxis sorting techniques also take their inspiration from natural selection during fertilization. On their journey to the egg, sperm cells encounter flows and viscoelastic fluids. It is believed that these conditions allow selection of the fittest sperms, i.e., the ones that are able to swim against the flow (= positive rheotaxis) and navigate in highly viscous fluids. Some microfluidic systems try to incorporate these principles one way or another to select highly motile sperm with high DNA integrity. One of the first, and certainly the most famous, microfluidic chips developed to sort sperm cells using laminar flows was invented by Cho et al. [12], [13] and was FDA approved in 2016 [14]. This system is illustrated in figure 5 and is driven passively with no external equipment. Initially made in PDMS, this design is now fabricated in COP thermoplastics which are more suitable for commercialization than PDMS as a large number of chips can be produced by injection molding. In this chip, two parallel streams of fluids, one containing the semen sample and one the buffer, flow at different speeds.
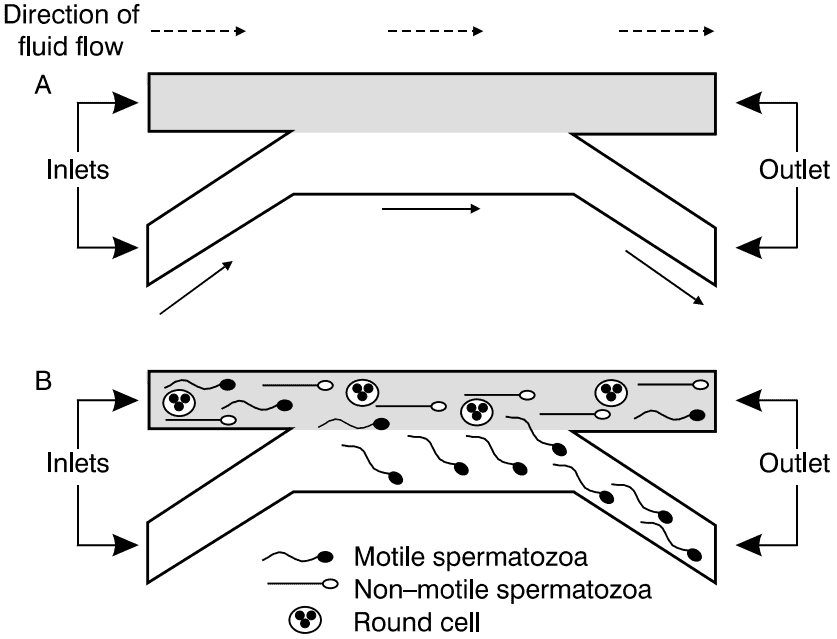
Figure 5 – Passively driven microfluidic device for sperm sorting based on motility [13]. A) Represents the flow direction and B) illustrates the sperm cells crossing streams.
Only highly motile sperm are able to cross the streams and swim to the buffer medium, thus allowing the retrieval of motile spermatozoa from this stream. More recently, a microfluidic system for sperm selection was developed based on this same cross-stream ability but this time, with flow rates controlled externally by syringe pumps and flow rate ratios varied depending on the semen sample viscosity. Flow-based systems improve the viability and retrieval rate of sperm cells and minimize DNA damage when compared to conventional techniques (swim up and centrifugation-based). Other microfluidic systems use the sperm’s rheotactic behavior to perform the sorting [15]–[17]. At very low flow rates, under a certain threshold, alive and motile spermatozoa will exhibit positive rheotaxis when placed in a flow. By tuning the flow speed, researchers were able to select highly motile cells with normal morphology and improve the global motility parameters of the sorted samples [18]. However, few studies take into account viscosity gradients or viscoelastic properties of the buffer in their sorting strategies.
Microfluidics for sperm sorting: gender-based selection
This sorting strategy is most useful to the dairy industry and to wildlife conservation. The most famous sex sorting method for sperm cells is flow cytometry, an expensive and damaging technique to the cells but which is still the most effective one on the market. Some alternatives based on microfluidic technology exist, such as the one developed by the company Engender which uses photonic technology coupled with a flow-based chip to sort X- and Y-bearing sperm cells. Because X- and Y-bearing sperm differ by 4% of their DNA content but also by their size, by using a pulse of light they are able to selectively move Y-bearing or X-bearing sperm cells from one fluid stream to the other. Recently, a group demonstrated the sex sorting ability of their microfluidic chip using dielectrophoresis (DEP) [19].
Microfluidics for sperm sorting: conclusion
The number of people in need of ARTs is growing and about half of infertility cases are due to male factors. Current technologies for sperm selection are usually time consuming, damaging to the sperm quality and integrity, low retrieval yields. Microfluidic systems have an important role to play in the democratization of sperm sorting technologies as well as improving the results of current sorting techniques. Although no studies yet show a significantly better outcome of IVF with sperm cells selected with a microfluidic sperm sorter, results are very promising and the ongoing research is likely to lead to a new paradigm in sperm sorting technologies.
![]() |
Review done thanks to the support of the ActiveMatter H2020-MSCA-ITN-2018-Action “Innovative Training Networks”, Grant agreement number: 812780 Author: Audrey Nsamela, PhD candidate |
![]() |
References
- S.M. Knowlton, M. Sadasivam, and S. Tasoglu, “Microfluidics for sperm research,” Trends Biotechnol., vol. 33, no. 4, pp. 221–229, 2015
- G. D. Smith, A. M. da Rocha, and L. Keller, “Microfluidics for Sperm Selection,” in Non-Invasive Sperm Selection for In Vitro Fertilization, A. Agarwal, E. Borges, and A. S. Setti, Eds. New York, NY: Springer New York, 2015, pp. 51–58.
- J. K. O’Brien, K. J. Steinman, and T. R. Robeck, “Application of sperm sorting and associated reproductive technology for wildlife management and conservation,” Theriogenology, vol. 71, no. 1, pp. 98–107, 2009
- Y. H. Lu, C. Y. Lu, and D. J. Yao, “Low DNA damage sperm sorting with varied viscosities in microfluidic chip,” Proc. IEEE Int. Conf. Micro Electro Mech. Syst., vol. 2018-Janua, no. January, pp. 1130–1133, 2018
- N. Kashaninejad, M. J. A. Shiddiky, and N. T. Nguyen, “Advances in Microfluidics-Based Assisted Reproductive Technology: From Sperm Sorter to Reproductive System-on-a-Chip,” Adv. Biosyst., vol. 2, no. 3, pp. 1–21, 2018
- R. Samuel, H. Feng, A. Jafek, D. Despain, T. Jenkins, and B. Gale, “Microfluidic-based sperm sorting & analysis for treatment of male infertility,” Transl. Androl. Urol., vol. 7, no. I, pp. S336–S347, 2018
- Y.-J. KO, J.-H. MAENG, B.-C. LEE, S. LEE, S. Y. HWANG, and Y. AHN, “Separation of Progressive Motile Sperm from Mouse Semen Using On-chip Chemotaxis,” Anal. Sci., vol. 28, no. 1, p. 27, 2012
- Y. J. Ko, J. H. Maeng, S. Y. Hwang, and Y. Ahn, “Design, Fabrication, and Testing of a Microfluidic Device for Thermotaxis and Chemotaxis Assays of Sperm,” SLAS Technol., vol. 23, no. 6, pp. 507–515, 2018
- A. Karbalaei and H. J. Cho, “Microfluidic devices developed for and inspired by thermotaxis and chemotaxis,” Micromachines, vol. 9, no. 4, 2018
- S. Boryshpolets, S. Pérez-Cerezales, and M. Eisenbach, “Behavioral mechanism of human sperm in thermotaxis: A role for hyperactivation,” Hum. Reprod., vol. 30, no. 4, pp. 884–892, 2015
- S. Pérez-Cerezales et al., “Sperm selection by thermotaxis improves ICSI outcome in mice,” Sci. Rep., vol. 8, no. 1, p. 2902, Dec. 2018
- B. S. Cho, T. G. Schuster, X. Zhu, D. Chang, G. D. Smith, and S. Takayama, “Passively Driven Integrated Microfluidic System for Separation of Motile Sperm,” Anal. Chem., vol. 75, no. 7, pp. 1671–1675, Apr. 2003
- T. G. Schuster, B. Cho, L. M. Keller, S. Takayama, and G. D. Smith, “Isolation of motile spermatozoa from semen samples using microfluidics,” Reprod. Biomed. Online, vol. 7, no. 1, pp. 75–81, 2003
- K. Shirota et al., “Separation efficiency of a microfluidic sperm sorter to minimize sperm DNA damage,” Fertil. Steril., vol. 105, no. 2, pp. 315-321.e1, 2016
- M. Zaferani, S. H. Cheong, and A. Abbaspourrad, “Rheotaxis-based separation of sperm with progressive motility using a microfluidic corral system,” Proc. Natl. Acad. Sci. U. S. A., vol. 115, no. 33, pp. 8272–8277, 2018
- B. Hwang, D. Lee, S. J. Hwang, J. H. Baek, and B. Kim, “Rheotaxis Based High-Throughput Motile Sperm Sorting Device,” Int. J. Precis. Eng. Manuf., vol. 20, no. 6, pp. 1037–1045, 2019
- M. Yaghoobi, M. Azizi, A. Mokhtare, and A. Abbaspourrad, “Progressive sperm separation using parallelized, high-throughput, microchamber-based microfluidics,” bioRxiv, 2020
- B. Zhang, T. L. Yin, and J. Yang, “A novel microfluidic device for selecting human sperm to increase the proportion of morphologically normal, motile sperm with uncompromised DNA integrity,” Anal. Methods, vol. 7, no. 14, pp. 5981–5988, 2015
- T. Wongtawan, N. Dararatana, C. Thongkittidilok, S. Kornmatitsuk, and B. Oonkhanond, “Enrichment of bovine X-sperm using microfluidic dielectrophoretic chip: A proof-of- concept study,” Heliyon, vol. 6, no. 11, p. e05483, 2020
- H. Chang, B. J. Kim, Y. S. Kim, S. S. Suarez, and M. Wu, “Different Migration Patterns of Sea Urchin and Mouse Sperm Revealed by a Microfluidic Chemotaxis Device,” PLoS One, vol. 8, no. 4, p. e60587, Apr. 2013
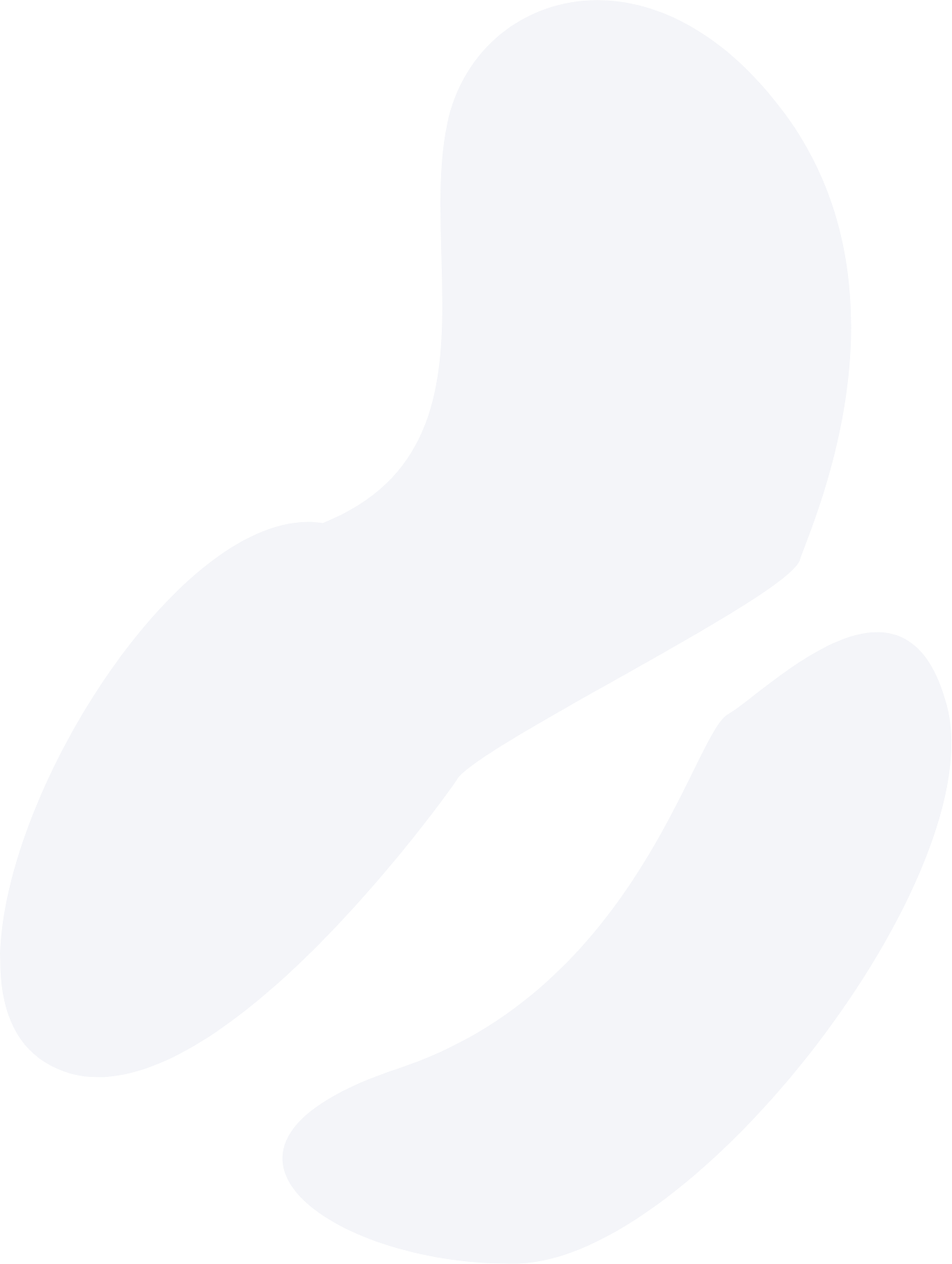
Microfluidics knowledge
Do you want tips on how to best set up your microfluidic experiment? Do you need inspiration or a different angle to take on your specific problem? Well, we probably have an application note just for you, feel free to check them out!